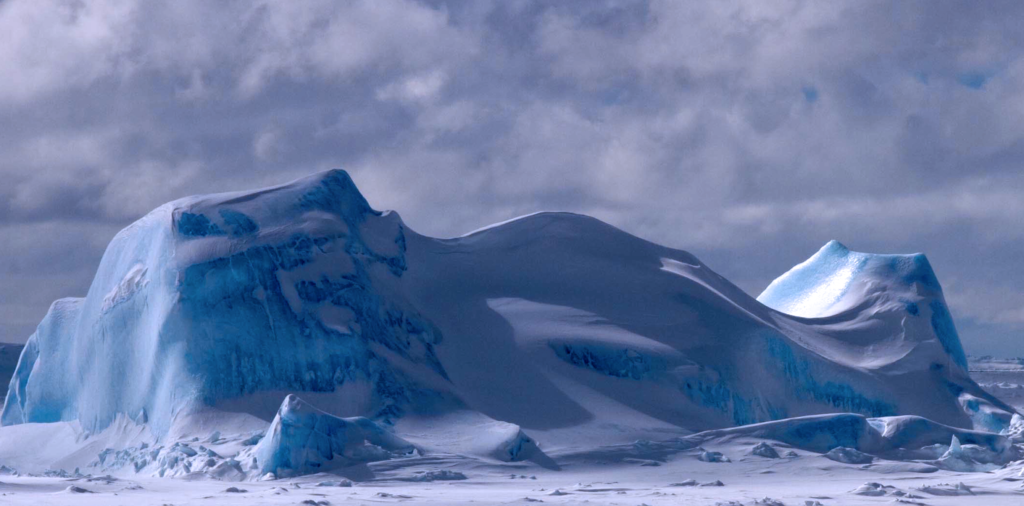
In the 2004 disaster movie “The Day After Tomorrow” New York City suddenly finds itself engulfed in thick ice within the matter of days after a series of superstorms hit the East coast. Apparently these storms were triggered by global warming, which paradoxically causes extreme cooling in New York. Cue Jake Gyllenhaal to come to the rescue. Sounds crazy, right? Well, fear not, it is indeed complete hogwash. But the basic premise of the movie, that a major reorganization of the ocean circulation can make some places on Earth much warmer while other places become much colder, is basically right. In the surface 200 m or so of the ocean currents are driven by wind, making waves that can cause a lot of havoc along the Jersey Shore when a stiff Nor’easter blows. But in the rest of the ocean—and there’s another 3.4 km to go before reaching the seafloor—it is tiny differences in temperature and salinity (and therefore density) that sets currents into motion. That’s why a thermometer and a salinometer are the two most important instruments for a physical oceanographer. With just those two measurements we can follow the water through all the major ocean basins. This constant movement of deep currents brings warm water from the tropics to the higher latitudes and cold water back towards the equator, a process that Wally Broecker at Lamont termed “the ocean conveyor belt”. And it is this heat transport that prevents New York from turning into a frozen tundra. So now lets talk about the 2000 flick “The Perfect Storm”… or maybe let’s not. Although it does have George Clooney in it.
The vastness and complexity of the ocean makes it difficult to sample and consequently limits our understanding of how it operates and its future trajectory. Observational technologies, such as satellite infrared and ocean color imagery, moorings, shore-based high frequency radars, profiling floats, and autonomous underwater vehicles, are vital for scientists and decision-makers to better understand ocean processes. Researchers in our department are involved in projects in the coastal waters off New Jersey and around the world where operational observatories are used to collect real-time data for adaptive sampling. With support from U.S. federal and state agencies, industry partners, private foundations, and foreign countries, we collaborate with other national and international research groups and commercial companies to develop new technologies for ocean sampling and tackle questions related to climate variability and long-term climate change. Data generated through our ocean observing efforts is used as a tool for undergraduate and graduate education.
Ocean Observation Faculty
Mountains washing into the sea are frequently invoked in music, art and literature. But these allegories omit the connection that links these end members: Estuaries. Look for a moment at your local river and you will witness the inexorable flow of water towards the sea and with it sediment, minerals, carbon and whatever else washes off the land and whatever we humans discharge into it. But the sea, heavily laden with salt, slumps underneath the river water and paradoxically flows upstream until it meets the river for battle on its own ground in the estuary. This convergence traps materials and accumulates food for the ecosystem, and supplies sediment to the marshes. The interplay between fresh and salt waters is agitated by tides and topography, which together make estuaries amongst the most dynamic and fascinating marine systems on earth.
Estuaries are also the most productive ecosystems on earth, and yet with 75% of the world’s population living in their watersheds, they are also the most impacted by humans. Thus we study them from a framework built on classical physics to ask: What drives their circulation? What controls the fate of material discharged into them? How they will respond to climate change and to human activities within their basin?
To better understand an estuary, we take its temperature and measure how fast it moves and swirls, the amount of salt flowing in its veins, and the amount of material that its flow carries. We stick scientific instruments into the water from a boat or affixed to a buoy that we hope haul in one day full of data. Sometimes we even dye the water red or yellow to visualize and quantify these beautifully complex mixing processes. We hold these data up against simple theories and complex computer models to advance our understanding of how estuaries work. We join forces with other marine and terrestrial scientists to understand how the physics of estuaries shape the marine ecology and how future change might alter their ecosystem and aesthetic value. This deeper understanding has vast societal implications such as predicting if a city will continue to be able to withdraw water for drinking in the face of sea-level rise and channel dredging or to determine the amount of industrial discharge a system can withstand without being compromised. Finally, this river water, modified by these estuarine processes, eventually debouches to the sea. But this story involves a discussion of coastal processes an equally fascinating and important topic that we save for a later day.
Coastal & Estuarine Processes Faculty
Polar regions play an integral role in regulating the Earth’s climate and have a major influence on biogeochemical cycles. Both the Arctic and Antarctic are particularly vulnerable to climate change. The loss of sea ice and permafrost and the collapse of glaciers as a result of climate change will impact the rich diversity of biota in these ecosystems as well as sea level rise. Our department has a very active polar research program, supported by multiple NSF grants, and involving many students from various disciplines. Rutgers scientists are engaged in both Arctic and Antarctic research using both regional and global climate models and via large collaborative efforts including GEOTRACES and the Palmer Long Term Ecological Research program, and utilize fieldwork opportunities in these regions to enrich undergraduate and graduate education.
Polar Research Faculty
The study of air-sea interactions is focused on how the atmosphere and ocean affect each other through the exchange of heat, momentum, and water across the air-sea interface. In general, the atmosphere receives heat from the ocean, thus affecting the atmospheric circulation and surface winds, which in turn generate ocean currents and the large scale ocean circulation. The spatial range of interactions spans from local heat fluxes to surface wave generation, formation of hurricanes, Arctic sea ice melt, and the nearly global scale of El Nino. Understanding how this tightly coupled system works is critical to our understanding of climate and how it might change in the future.
Air-Sea Interaction Faculty
The study of matter and its motion through space and time is essential to oceanography, with physical processes spanning the subcellular to macroscale over millisecond to annual frames. Marine phytoplankton have influenced our profoundly shaped our planet through the oxygenation of the Earth’s atmosphere and the removal of fixed CO2 to the deep ocean by photosynthesis and sinking, a process called the ‘biological pump’. The process ultimately derives from their innate ability to capture protons and electrons from water and transport them across membranes in photosystems. These types of subcellular biophysical interactions drive the function and evolution of proteins, biochemical processes and biological cell systems. At the same time, the physical motion of water is integral to marine life, affecting organism form and function through ecological interactions and transport processes. Water movement provides communication signals and behavioral cues to small plankton and larvae. Likewise, fluid forces affect the mechanics and energetics of swimming and the ability of bottom-dwellers to remain attached to the seabed. Turbulence, waves, and currents carry planktonic organisms and determine how they are distributed in space and time. Collectively, these water motions are a selective force, driving behavior, body shapes, and other adaptations that help organisms thrive. Ongoing departmental research investigates biophysical form and function of photosynthesis and related metabolic pathways, as well as how organism-flow interactions affect motion sensing and swimming behavior, energetics, larval transport and settlement, and gene flow among marine populations.
Environmental Biophysics Faculty
Maxim Gorbunov
Thomas Grothues (RUMFS)
Sediment (clay, silt, sand and gravel) is needed to create and maintain ocean and coastal depositional landforms and habitats, such as bars, beaches, dunes and salt marshes. Sediment transport often follows clearly defined paths (channels) but can occur over broad areas, especially during storms, when rates of sediment movement can be greatly accelerated. Transport away from a location (erosion) is often considered a negative effect, although this erosion often supplies sediment to another location (accretion) that can help maintain landforms and habitats. Flora and fauna are adapted to these changes in sediment transport, but humans are likely to view these changes as a sign of instability. Human actions can remove sediment deposits or sever pathways of sediment transport, requiring the use of mechanical equipment to artificially create or maintain depositional landforms and habitats.