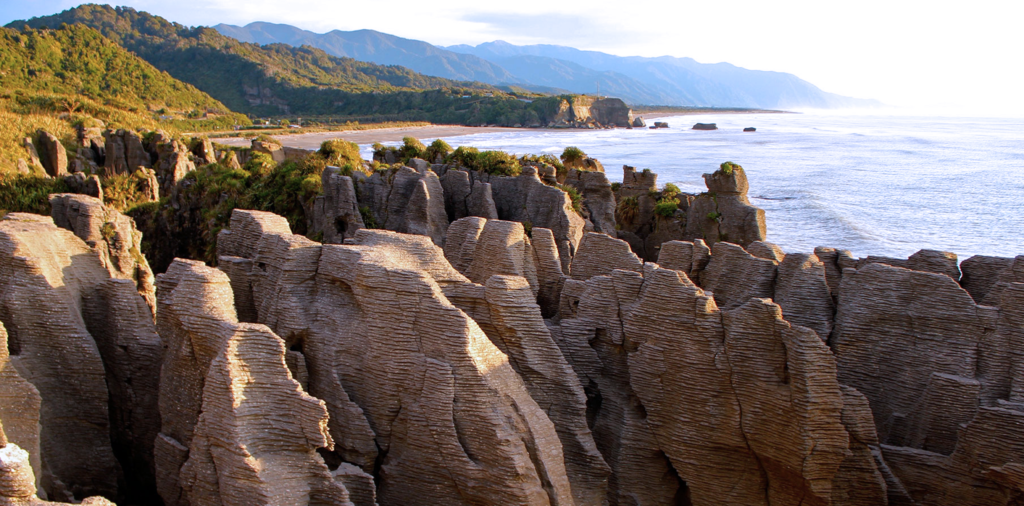
As the saying goes: ”What happens in Vegas stays in Vegas”. Well, turns out the ocean is not as good at keeping a secret. Most of what happens in the ocean ends up on the seafloor. As such, sediments are the long-term memory of the ocean, a window into what Earth looked like thousands or even millions of years back in time. From this muddy library we can learn about the rise and fall of the sea as polar ice sheets have come and gone. There were no thermometers when the dinosaurs roamed the Earth, but marine sediments can tell us that the planet was very much warmer 65 million years ago… that is, until that huge meteorite hit the Earth, causing the end of an era, and turning the Earth into an (nearly) unihabitable wasteland for the next few decades. And guess what, traces of that meteorite impact can be found in the thick sediment layers just off the New Jersey coast. In the deepest part of the ocean where there is no sediment cover, seawater is sucked into the seafloor along huge mountain ranges. As the seawater is heated in the crust, it extracts precious metals from the Earth, and where the hot seawater re-emerges it gives life to some of the most intriguing life forms that populate hydrothermal vents. Maybe it is the seafloor near such hydrothermal vents that holds the secret to how life on Earth began. And ironically, some ancient seafloor from the earliest part of Earth’s history can now be found in the desert of Nevada, just outside Vegas.
Reconstructions of former sea levels provide context to frame recent accelerations and refine future projections of sea-level rise. Sea-level reconstructions help refine model predictions of glacial-isostatic adjustment (GIA) and aid our understanding of the spatial and temporal response to climate change. Sea-level reconstructions from coastal sediments are relevant to varied disciplines including studies of coastal evolution and wetland development, ice-sheet history, paleoclimate and understanding the environmental context of human activity. Reconstruction from coastal sedimentary record also provide an archive of the occurrence of former earthquakes, tsunamis and tropical cyclones. Observational and instrumental records are too short (particularly for less frequent, high magnitude events) to provide a reliable basis for predicting future activity Therefore, coastal sedimentary records are a valuable tool to assess future risk.
Sea Level Change Faculty
Mountains washing into the sea are frequently invoked in music, art and literature. But these allegories omit the connection that links these end members: Estuaries. Look for a moment at your local river and you will witness the inexorable flow of water towards the sea and with it sediment, minerals, carbon and whatever else washes off the land and whatever we humans discharge into it. But the sea, heavily laden with salt, slumps underneath the river water and paradoxically flows upstream until it meets the river for battle on its own ground in the estuary. This convergence traps materials and accumulates food for the ecosystem, and supplies sediment to the marshes. The interplay between fresh and salt waters is agitated by tides and topography, which together make estuaries amongst the most dynamic and fascinating marine systems on earth.
Estuaries are also the most productive ecosystems on earth, and yet with 75% of the world’s population living in their watersheds, they are also the most impacted by humans. Thus we study them from a framework built on classical physics to ask: What drives their circulation? What controls the fate of material discharged into them? How they will respond to climate change and to human activities within their basin?
To better understand an estuary, we take its temperature and measure how fast it moves and swirls, the amount of salt flowing in its veins, and the amount of material that its flow carries. We stick scientific instruments into the water from a boat or affixed to a buoy that we hope haul in one day full of data. Sometimes we even dye the water red or yellow to visualize and quantify these beautifully complex mixing processes. We hold these data up against simple theories and complex computer models to advance our understanding of how estuaries work. We join forces with other marine and terrestrial scientists to understand how the physics of estuaries shape the marine ecology and how future change might alter their ecosystem and aesthetic value. This deeper understanding has vast societal implications such as predicting if a city will continue to be able to withdraw water for drinking in the face of sea-level rise and channel dredging or to determine the amount of industrial discharge a system can withstand without being compromised. Finally, this river water, modified by these estuarine processes, eventually debouches to the sea. But this story involves a discussion of coastal processes an equally fascinating and important topic that we save for a later day.
Coastal & Estuarine Processes Faculty
About 4 billion people live within 100 km of the earth’s 356,000 km of coastline and these intense concentrations of population, economic production, and static infrastructure are vulnerable to changes in sea level brought about by climate change, storms or earthquakes. Coastal risk assessment and hazard mitigation requires datasets on centennial and millennial temporal scales to capture natural variability and multiple occurrences of the largest, but least frequent events. Coastal sediments from low-energy depositional environments archive geologic evidence of past sea-level change, palaeoearthquakes, tsunamis, and storms.
Coastal Hazards Faculty
A biogeochemical cycle describes a pathway by which a chemical substance (oxygen, carbon, nitrogen) moves through both biotic and abiotic compartments of Earth. Elements, chemical compounds, and other forms of matter are passed from one organism to another and from one part of the biosphere or geosphere to another through biogeochemical cycles. The term “biogeochemical” tells us that biological, geological and chemical factors are all involved. The circulation of essential nutrients like carbon, oxygen, nitrogen, phosphorus, calcium, iron and water etc. through the biological and physical world are known as biogeochemical cycles.
Biogeochemical Cycles Faculty
On February 17, 1977, pilot Jack Donnelly and geologists Jack Corliss and Jerry van Andel were surveying an area of the seafloor along the Galapagos Rift. The pilot and the two scientists were navigating aboard the deep-submergence vehicle Alvin at a depth of 2500 meters when they entered a bizarre, 20-meter-wide oasis of life populated by giant white clams clustered around shimmering, milky fluids escaping from cracks in the seafloor. Alvin’s heat sensor recorded 16°C in the cracks, a relative mild temperature compared to the frigid 2°C of the deep ocean. “Isn’t the deep ocean supposed to be a desert? Well, there’s all these animals down here…”, Jack Corliss announced over the acoustic telephone. While temperature anomalies had been recorded earlier along mid-oceanic ridges, and the existence of hydrothermal circulation at the bottom of the ocean had long been hypothesized, Alvin dive 713 went down in history as the official discovery of deep-sea hydrothermal vents.
Deep at the bottom of the ocean, geology, chemistry and biology boil together at hydrothermal vents. Magma near the surface makes new oceanic crust and also heats rock and seawater to create a chemical brew that alters the chemistry of the ocean. These hydrothermal fluids are laden in reduced chemical species that provide energy and carbon to chemosynthetic bacteria that support rich communities of invertebrates in the otherwise seemingly barren abyss. Giant tubeworms, shrimp with no eyes, hairy snails, and yeti crabs have found ways to farm, harvest and collaborate with these microbes. But these chemical and biological oases can be transient. The magma that starts the whole process can erupt and wipe the slate clean, or recede and starve the vent. Rutgers scientists integrate field and laboratory work to study the physiology, ecology and evolution of the unique microbial and animal communities at hydrothermal vents and to quantify the contribution of marine hydrothermal systems to global biogeochemical cycles. Rutgers researchers dive to the seafloor using deep-diving submersibles, remotely operated and autonomous vehicles to explore and sample hydrothermal vents around the world’s oceans.
Acoustic Imaging & Quantifying Seafloor Hydrothermal Vent Flow
Hydrothermal Systems Faculty
Sediment (clay, silt, sand and gravel) is needed to create and maintain ocean and coastal depositional landforms and habitats, such as bars, beaches, dunes and salt marshes. Sediment transport often follows clearly defined paths (channels) but can occur over broad areas, especially during storms, when rates of sediment movement can be greatly accelerated. Transport away from a location (erosion) is often considered a negative effect, although this erosion often supplies sediment to another location (accretion) that can help maintain landforms and habitats. Flora and fauna are adapted to these changes in sediment transport, but humans are likely to view these changes as a sign of instability. Human actions can remove sediment deposits or sever pathways of sediment transport, requiring the use of mechanical equipment to artificially create or maintain depositional landforms and habitats.