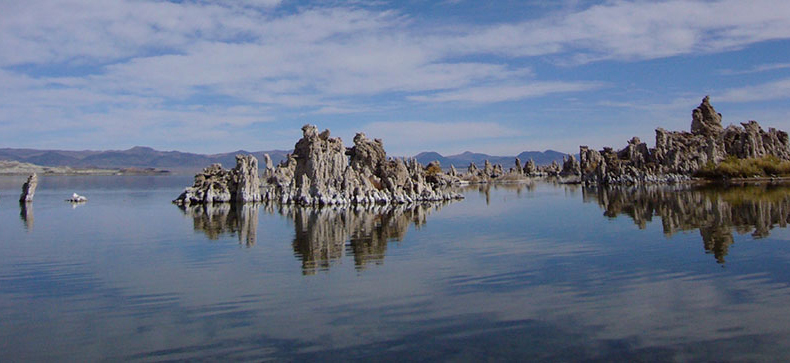
The seafloor is the final resting place for much of the materials washed into the ocean from land, for minerals forming in the ocean, and for detritus from organism that once populated the sunlit surface ocean. But the exchange between the seafloor and the overlying water column is not a one-way process. Once the material settles on the seafloor it is often subjected to intense biological reworking and recycling. Some of this recycled substrate makes it back into the water column, and if it is picked up by upwelling currents it might even be returned to the sunlight surface to once again supply nutrients to the biota, completing the cycle. The exchange the between the water column and the seafloor is especially important in the shallow waters of the highly productive coastal oceans. In our department we study the environmental controls of this bi-directional exchange process and linkages between biology and chemistry.
Benthic-Pelagic Coupling Faculty
Our students hard at work about the R/V Sharp filtering water to collect organic carbon samples show their sense of humor and spell out TOC (total organic carbon) an important part of the marine carbon cycle.
Carbon is the fundamental element of life. The carbon cycle is the interlinked pathways by which carbon transitions between being an inorganic component of the environment and the fundamental structural component of all things living. Through biologic uptake via photosynthesis carbon is transformed from carbon dioxide to organic carbon compounds (better known as living organisms) and then through respiration, death, and fossil fuel burning it is returned back to carbon dioxide. These processes are at the heart of both the carbon cycle and the anthropogenic alterations of the cycle. Less well known is the importance of the biological uptake of carbon dioxide by marine organisms to form calcium carbonate shells that are an important arm of both the carbon cycle and balancing ocean acidification. Understanding how carbon is passed from one organism to another and from the biosphere to the geosphere is a research focus of a number of Rutgers Marine and Coastal Science faculty.
Carbon Cycling Faculty
How can we know the surface ocean temperature near the equator during the last ice age? This is the fundamental quandary of paleoceanography: how to reconstruct environmental variables that can no longer be measured directly. Proxies are chemical, physical or biological cues that correlate with the parameter of interest (e.g., temperature, pH, nutrient status) in a predictable fashion, and that are well preserved in the geological record over thousands or even millions of years. New proxies are constantly developed, and existing proxies are refined and improved. Researchers in our department have a strong track record in the development, calibration and validation of new and existing proxies. These proxies help us understand how the Earth’s surface environment has changed through time, and what processes and feedbacks have driven this environmental change.
Proxy Development Faculty
On February 17, 1977, pilot Jack Donnelly and geologists Jack Corliss and Jerry van Andel were surveying an area of the seafloor along the Galapagos Rift. The pilot and the two scientists were navigating aboard the deep-submergence vehicle Alvin at a depth of 2500 meters when they entered a bizarre, 20-meter-wide oasis of life populated by giant white clams clustered around shimmering, milky fluids escaping from cracks in the seafloor. Alvin’s heat sensor recorded 16°C in the cracks, a relative mild temperature compared to the frigid 2°C of the deep ocean. “Isn’t the deep ocean supposed to be a desert? Well, there’s all these animals down here…”, Jack Corliss announced over the acoustic telephone. While temperature anomalies had been recorded earlier along mid-oceanic ridges, and the existence of hydrothermal circulation at the bottom of the ocean had long been hypothesized, Alvin dive 713 went down in history as the official discovery of deep-sea hydrothermal vents.
Deep at the bottom of the ocean, geology, chemistry and biology boil together at hydrothermal vents. Magma near the surface makes new oceanic crust and also heats rock and seawater to create a chemical brew that alters the chemistry of the ocean. These hydrothermal fluids are laden in reduced chemical species that provide energy and carbon to chemosynthetic bacteria that support rich communities of invertebrates in the otherwise seemingly barren abyss. Giant tubeworms, shrimp with no eyes, hairy snails, and yeti crabs have found ways to farm, harvest and collaborate with these microbes. But these chemical and biological oases can be transient. The magma that starts the whole process can erupt and wipe the slate clean, or recede and starve the vent. Rutgers scientists integrate field and laboratory work to study the physiology, ecology and evolution of the unique microbial and animal communities at hydrothermal vents and to quantify the contribution of marine hydrothermal systems to global biogeochemical cycles. Rutgers researchers dive to the seafloor using deep-diving submersibles, remotely operated and autonomous vehicles to explore and sample hydrothermal vents around the world’s oceans.
Acoustic Imaging & Quantifying Seafloor Hydrothermal Vent Flow
Hydrothermal Systems Faculty
Mountains washing into the sea are frequently invoked in music, art and literature. But these allegories omit the connection that links these end members: Estuaries. Look for a moment at your local river and you will witness the inexorable flow of water towards the sea and with it sediment, minerals, carbon and whatever else washes off the land and whatever we humans discharge into it. But the sea, heavily laden with salt, slumps underneath the river water and paradoxically flows upstream until it meets the river for battle on its own ground in the estuary. This convergence traps materials and accumulates food for the ecosystem, and supplies sediment to the marshes. The interplay between fresh and salt waters is agitated by tides and topography, which together make estuaries amongst the most dynamic and fascinating marine systems on earth.
Estuaries are also the most productive ecosystems on earth, and yet with 75% of the world’s population living in their watersheds, they are also the most impacted by humans. Thus we study them from a framework built on classical physics to ask: What drives their circulation? What controls the fate of material discharged into them? How they will respond to climate change and to human activities within their basin?
To better understand an estuary, we take its temperature and measure how fast it moves and swirls, the amount of salt flowing in its veins, and the amount of material that its flow carries. We stick scientific instruments into the water from a boat or affixed to a buoy that we hope haul in one day full of data. Sometimes we even dye the water red or yellow to visualize and quantify these beautifully complex mixing processes. We hold these data up against simple theories and complex computer models to advance our understanding of how estuaries work. We join forces with other marine and terrestrial scientists to understand how the physics of estuaries shape the marine ecology and how future change might alter their ecosystem and aesthetic value. This deeper understanding has vast societal implications such as predicting if a city will continue to be able to withdraw water for drinking in the face of sea-level rise and channel dredging or to determine the amount of industrial discharge a system can withstand without being compromised. Finally, this river water, modified by these estuarine processes, eventually debouches to the sea. But this story involves a discussion of coastal processes an equally fascinating and important topic that we save for a later day.
Coastal & Estuarine Processes Faculty
Polar regions play an integral role in regulating the Earth’s climate and have a major influence on biogeochemical cycles. Both the Arctic and Antarctic are particularly vulnerable to climate change. The loss of sea ice and permafrost and the collapse of glaciers as a result of climate change will impact the rich diversity of biota in these ecosystems as well as sea level rise. Our department has a very active polar research program, supported by multiple NSF grants, and involving many students from various disciplines. Rutgers scientists are engaged in both Arctic and Antarctic research using both regional and global climate models and via large collaborative efforts including GEOTRACES and the Palmer Long Term Ecological Research program, and utilize fieldwork opportunities in these regions to enrich undergraduate and graduate education.
Polar Research Faculty
A biogeochemical cycle describes a pathway by which a chemical substance (oxygen, carbon, nitrogen) moves through both biotic and abiotic compartments of Earth. Elements, chemical compounds, and other forms of matter are passed from one organism to another and from one part of the biosphere or geosphere to another through biogeochemical cycles. The term “biogeochemical” tells us that biological, geological and chemical factors are all involved. The circulation of essential nutrients like carbon, oxygen, nitrogen, phosphorus, calcium, iron and water etc. through the biological and physical world are known as biogeochemical cycles.